Medicinal Chemistry and Chemical Biology of Misfolded Proteins
Protein stabilisation has been subject to intense research in recent years with the aim of developing novel therapeutic strategies that focus on restoring the functionality of misfolded proteins driving a wide range of diseases. Considerable success has been achieved in either correcting misfolding/stabilizing active but unstable proteins by use of pharmacological “chaperones”, or by preventing early degradation with inhibitors of the proteasome and ERAD components. We are developing small molecule chaperones targeting mutated proteins whose instability leads to diseases, to identify proof-of-concept pre-clinical candidates towards first-in-class therapeutics. Current systems under investigation include mutated forms of the tumour suppressor p53 (cancer) and Formylglycine Generating Enzyme (Multiple Sulfatase Deficiency).
1) Drugging p53 cancer mutants.
Background and importance: The tumour suppressor p53 is referred to as the ”guardian of the genome” due to its pivotal role in many critical cellular processes, including cell-cycle regulation, DNA repair and apoptosis. Dysregulation of its activity leading to impaired or abrogated p53 signalling is a hallmark of the estimated 18 million new cancer cases reported yearly worldwide, making the p53 pathway a well-validated although extremely challenging target for anticancer drug development. Inhibition of MDM2 has been pursued clinically for over a decade and still has not delivered an effective drug. With a few exceptions, other strategies based on p53 gene therapy have also known very limited success. More recently, the progression of the small molecules APR-246 and PC14586 for the treatment of mutant p53 tumours has generated huge interest and excitement, and highlighted the clinical feasibility of small molecule-mediated reactivation of mutant p53 for therapy development.
p53 is inactivated directly by mutation in ca. 50% of all cancers, with the majority of point mutations arising in its DNA-binding domain (DBD, aa 94-292), in turn critically affecting its DNA-binding specificity (“contact mutants”) and/or thermal stability (“structural mutants”). An estimated one third of common p53 cancer mutants are temperature sensitive structural mutants, and undergo loss of function through rapid denaturation under physiological conditions (path A). Remarkably, a number of such unstable mutants display wild-type (WT) like conformation and residual transcriptional activity at sub-physiological (permissive) temperatures. This suggests that their stability and function can be rescued by small molecule pharmacological chaperones stabilising their structure by binding to their folded, functional form (path B). This provides a blueprint for developing novel classes of personalised drugs targeting a range of p53 mutants and restoring their tumour suppressor ability.

p53 DBD structure and mutations. A) Crystal structure of the WT p53 DBD (monomer) bound to a DNA response element. Tyrosine 220 is highlighted in red ; B) Relative missense mutation frequency (Y-axis) of p53 residues (X-axis) in human cancer. Residue 220 is highlighted (red arrow). TAD: transactivation domain; PRR: proline rich region; p53C: p53 « core » DNA binding domain; TET: tetramerisation domain; CT: C-terminal domain; C) surface representation of WT p53 DBD (pdb 1UOL), close-up on residue Y220 (yellow); D) Extended surface crevice induced by the Y220C mutation (C220 shown in yellow).
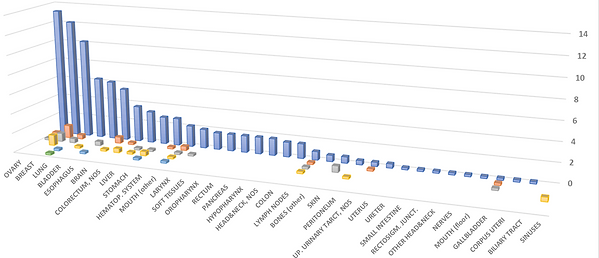
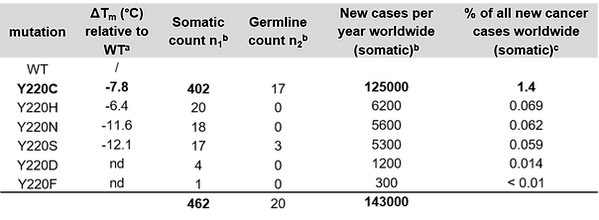
Top - Distribution of Y220X mutations across 35 types of cancers. X-axis: cancer type; Y-axis: rate (%); blue: Y220C; red: Y220H; grey: Y220N; yellow: Y220S; blue: Y220D; green: Y220F; Σ %i = 100%; N = 462 (see Table 1).
Bottom - Stability and frequency of p53 cancer mutation at codon 220. a) Thermostability of the four most frequent Y220X mutants relative to WT, measured by differential scanning fluorimetry using a stabilised quadruple mutant (QM) DBD (M133L/V203A/N239Y/N268D) which has successfully been employed previously for biophysical and structural studies of labile p53 structural mutants.(20,21,17) Taken from Joerger et al. 2020. b) Number of cases in the R20 release from the International Agency for Research in Cancer (IARC) database of p53 cancer mutations. N1 = 28866 for somatic and N2 = 1245 for germline datasets. Estimated based on the ratio of the somatic count and total number of reported somatic p53 cancer mutations in the IARC database, an estimated total of 18 million new cancer cases worldwide (2018), and an estimated overall p53 mutation rate of 50%. (n1/N1).18000000 x 0.5; c) Estimated based on the ratio of the somatic count and total number of reported somatic p53 cancer mutations in the IARC database (n1/N1).
Our research focuses on the identification and medicinal chemistry optimisation of small molecule pharmacological chaperones. Such small molecules act as molecular “plasters”, stabilising and rescuing the function of misfolded p53 structural mutants encountered in diverse types of cancers. One such mutant is p53-Y220C: Surface exposed tyrosine Y220 is the seventh most frequently mutated residue in human cancer, and Y220C alone is overall the ninth most frequent p53 cancer mutation. Mutation of residue Y220 to C creates a well-defined pocket on the surface of the p53 DBD that reduces its stability by ca. 4 kcal/mol (~ 5-7°C). It is estimated that a small molecule-based p53-reactivating drug, stabilising the p53-Y220C mutant, could provide an effective treatment for >120,000 new cases of cancer per annum worldwide. We and our collaborators have developed several lead molecules binding to the Y220C pocket with low μM affinity (Kd) in vitro, stabilizing the protein by up to 3-4 °C. We recorded high-resolution X-ray co-crystal structures for structure based optimisation, and derived structure-activity relationships (SAR) from cell biology and biophysical assays. Representative lead MB725 i) stabilises p53-Y220C in vitro in a concentration dependent manner; ii) binds potently to the Y220C pocket (Kd = 4 μM, 1H/15N HSQC NMR and ITC); iii) shows dose dependent, selective viability reduction in diverse p53-Y220C cancer cell lines, while maintaining comparatively low toxicity in the same concentration range in wt-p53 cancer lines, along with in house, CRISPR engineered p53-KO cells; iv) Reduced Y220C cell viability correlates with enhanced and selective transcription of a range of p53 target genes, including BTG2, p21, PUMA, FAS, TNF, and TNFRSF10B, which promote apoptosis or cell cycle arrest, suggesting compound-mediated transcriptional activation of p53-Y220C (qPCR study of 84 genes under p53 control). Current leads are being optimised in our laboratory, with the aim of delivering candidate molecules with suitable properties, potencies and toxicity profiles for (pre)clinical studies.
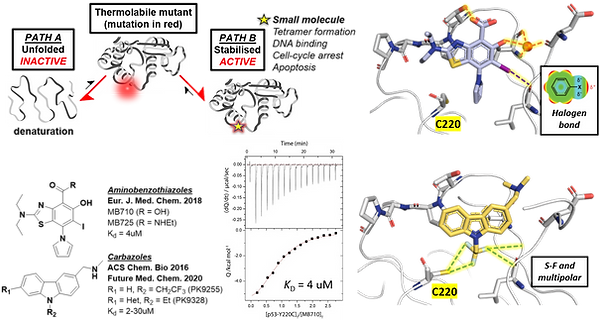
2) Multiple Sulfatase Deficiency (MSD, aka Austin's Disease).
Background and importance: MSD is an extremely rare and inherited lysosomal storage disorder (LSD), which is fatal and currently untreatable. MSD is characterized by deficient activities of all 17 sulfatase enzymes in patients, resulting in the accumulation of diverse sulfatides and glycosaminoglycans to toxic levels. In normal conditions, sulfatases require activation in the endoplasmic reticulum (ER) by post-translational modification of a conserved cysteine residue in their active site to the catalytic Cα-formylglycine (FGly) residue, and catalysed by the formylglycine-generating enzyme (FGE), the gene product of the SUMF1 gene. SUMF1 mutations that impair FGE function are a hallmark of MSD and were identified as its molecular basis. These MSD-causing mutations are hypomorphic, so that nearly all mutations lead to a partial loss of activity whereas a complete loss of FGE function is embryonically lethal in mice. From the ca. 50 mutations in the SUMF1 gene described, two-thirds are missense mutations that result in unstable and catalytically impaired FGE variants that, while retaining some residual activity, are rapidly degraded in cells, thus causing MSD. Importantly, the manifestation of severity in MSD is linked to residual functionality of these FGE variants, directly impacting the levels of activated sulfatases downstream. Additionally, extensive studies of the biochemical properties of FGE-mutants from patients’ cells have shown that the residual activity of FGE is dependent on its intracellular stability. In other words, the apparent low in vivo residual activity of misfolded FGE is the result of its accelerated degradation via the ERAD pathway. These findings put MSD into the category of protein misfolding diseases for which rescue of the residual activity serves as a window for therapeutic intervention.
Our research focuses on the development of candidate therapeutic agents for MSD acting by rescuing the activity of mutated FGE and ultimately the downstream sulfatases. We are using an amalgam of fragment screening, drug repurposing, and HTVS to identify lead molecules acting via several modes of action, including (i) upregulation of FGE expression, (ii) stabilisation by direct binding of a small molecule (similarly to p53 reactivators above) or via drug mediated expression of a chaperoning cellular partner, or (iii) escape of the cellular ER quality control.
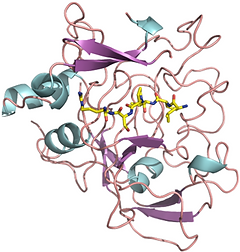
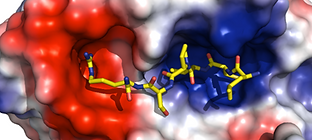
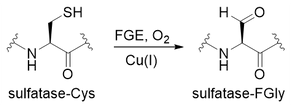
2AIK (FGE in complex with ArA peptide LCTPSRA)

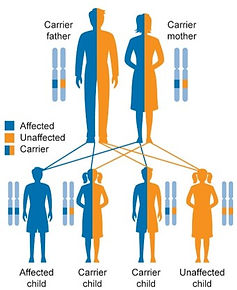
< 100 known cases worldwide
Prognosis: av. LE 10 yo
1/1000 silent carriers
Ca. 50 known mutations
2/3 missense

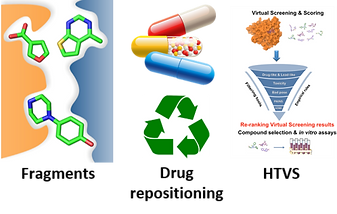